At least 20,000 unique proteins labor at a dizzying array of tasks in cells, collectively enabling our tissues, organs and bodies to function. Many of these proteins also play important roles in diseases, so drug hunters search for ways to influence their activity. But entire classes of proteins have proven difficult to target, leaving some diseases untreatable.
Researchers at Novartis and the University of California, Berkeley, seek to tackle these so-called “undruggable” proteins. A new Novartis-Berkeley Center for Proteomics and Chemistry Technologies in the US will focus on the portion of the proteome that dodges conventional small molecules, the standard ammunition of drug hunters. These elusive proteins seem to be missing the indentations – or pockets – that such small molecules need to bind and interrupt the protein’s work. Collaborators from industry and academia will use emerging technologies to identify previously hidden binding pockets on proteins as well as starting points for new therapeutics.
“One of the biggest challenges we face in treating human disease in the modern era is that most of the proteome is considered undruggable,” says Dan Nomura, director of the center and an associate professor of chemistry, of molecular and cell biology, and of nutritional sciences and toxicology at Berkeley. “The goal of the Novartis-Berkeley Center is to drug the undruggable.”
Based at Berkeley, the center will build on recent advances in a field called covalent chemoproteomics, Nomura’s specialty. Investigators from Novartis and Berkeley will use this new proteomics platform to interrogate elusive proteins, collaborating to test and apply the technology.
“We’re looking forward to learning how to use this platform from our colleagues in academia,” says John Tallarico, Head of Chemical Biology and Therapeutics at the Novartis Institutes for BioMedical Research (NIBR), the research arm of Novartis. “The technology has matured to the point that it can be applied to drug discovery, and we’re excited to collaborate on some proof-of-concept projects.”
Whatever science, whatever new technology that has been reported, if it looks like it will be useful for drugging the 'undruggable,' that’s where we need to go.
John Tallarico, Head, Chemical Biology and Therapeutics, Novartis Institutes for BioMedical Research
The proof-of-concept projects will be carried out in conjunction with four Berkeley labs – Nomura’s, Chris Chang’s, Dean Toste’s and Tom Maimone’s – where advances in both chemistry and proteomics will be developed. The scientists hope to publish their findings and share their strategies with the scientific community in addition to spurring drug discovery programs.
Chemical biology at its core
The collaboration is a springboard for a broader effort at Novartis to expose scientists to new ideas and foster unconventional thinking with the goal of pursuing difficult drug targets. In 2016, NIBR formed the Chemical Biology and Therapeutics group – comprising hundreds of researchers ranging from chemists to biologists to computer scientists – to break down silos between disciplines and encourage the teamwork required to hunt and attack challenging targets.
The group employs an approach called chemical biology, which involves creating and using new types of molecules to probe biological systems. The platform at the heart of the Novartis-Berkeley collaboration fits this mold. It exploits recent advances in chemistry to help scientists mine the proteome in the context of living cells.
The platform in action
Scientists start with a library of compounds capable of forming covalent bonds, a path that has seen a resurgence recently but is still far from routine. Conventional small molecule drugs – and compounds curated by pharmaceutical companies – typically interact with proteins through transient electrostatic, hydrophobic or π-effects rather than by forming permanent covalent bonds. Think of them as teenagers in short-term relationships.
In contrast, the Novartis-Berkeley collaborators will use compounds that form stable, long-term relationships. These compounds potentially interact with proteins that the other molecules miss or ignore, including proteins that are currently considered undruggable.
Each compound is applied to a cluster of cells, where it might bind to one or more proteins in the cells. The trick is to identify all of the binding partners.
“As the compound is irreversibly bound to the protein, it is much easier to figure out the identity of the protein and where it has interacted,” says Jeff McKenna, a senior investigator in Global Discovery Chemistry at NIBR who helped establish the collaboration. “That’s the beauty of this platform.”
After the compound is applied to the cluster, the researchers douse the cells with a chemical probe designed to react with the amino acid cysteine, a protein building block that can form covalent bonds. Unlike the initial compound, which only reacts with cysteines after nestling into a binding pocket that fits, the probe isn’t picky. It scours the proteome and forms covalent bonds with any free cysteines that haven’t already reacted with the compound.
Next, the researchers fish out all of the proteins that are bound to the probe, using a tag on it as a handle. Proteins that bound to the initial compound rather than to the probe are left behind.
Using a technique called mass spectrometry, scientists compile a list of proteins that they’ve fished from the cells. They compare the list with one from a control cluster of cells – doused with the probe but not the chemical – to determine which proteins are missing. These are the targets of the compound.
“We can identify new binding pockets within proteins and small molecules that fit the binding pockets in a single experiment,” explains Nomura.
“We’d love to start a massive data collection effort to see if we can find a small molecule for every protein in the proteome,” adds Tallarico. “These molecules could serve as starting points for drugs.”
The collaborators will also investigate how to convert the starting points into therapeutics. Although a given small molecule binds to a protein target, it doesn’t necessarily interrupt the protein’s work. In cases where the function of the protein has not been affected, the scientists will try hitching the small molecule to cellular machinery that destroys proteins, an approach called targeted protein degradation. The researchers also plan to experiment with novel covalent chemistries and modify the platform itself to access as many difficult proteins as possible in unique modes.
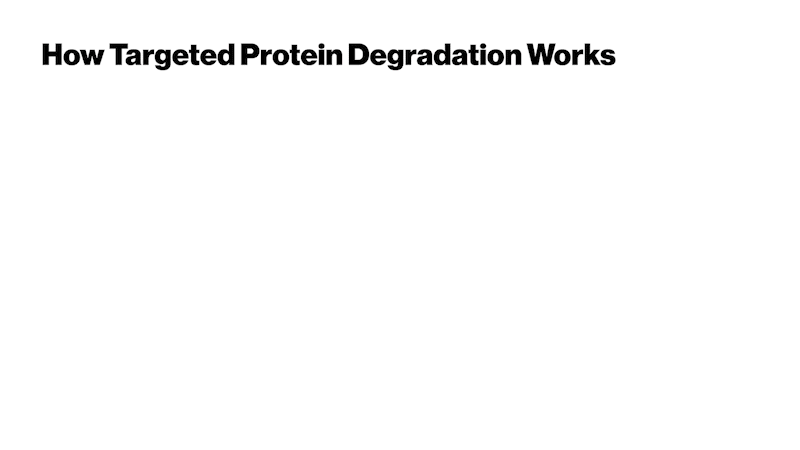