For some large and powerful new experiments in neurological disease, the tiny zebrafish’s extremely tiny brain is just the right size.
It’s small enough that researchers can watch the activity of single brain cells across an entire living brain.
But it’s large enough for the 3-centimeter-long fish to display social behaviors that may correlate with human conditions such as autism.
That makes the zebrafish an excellent model for studying patterns of activity in the brain linked to human health and disease, says Peixin Zhu, leader of the zebrafish research hub at the Novartis Institutes for BioMedical Research (NIBR). After all, humans and zebrafish share a lot of DNA. In fact, 84 percent of genes associated with human disease have a zebrafish counterpart.1 So Zhu’s team is working to establish big-data imaging of zebrafish brains and behaviors as a model for drug discovery.
“We’re imaging zebrafish brains and behaviors to lay a foundation for drug discovery in Neuroscience,” says Zhu.
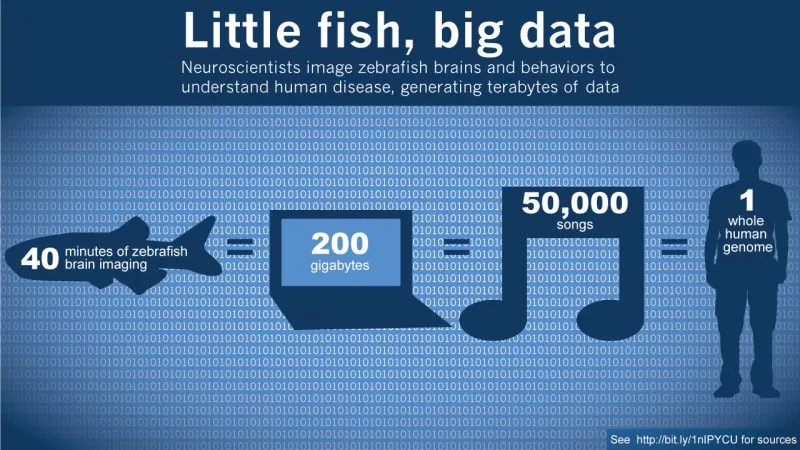
While the program is still in its early days, Zhu and his colleagues are starting to get intriguing hints about how, for example, modifying one gene implicated in human autism may change brain patterns and alter the body language of two male fish sharing a tank.
This information will complement the clues gathered in recent human genetic studies.
Hundreds of genes are associated with conditions such as autism and schizophrenia, but scientists don’t know exactly how these genes contribute to the conditions, individually or in combination. Zhu’s group is trying to map these genes to neural circuits and behaviors in the fish, working one gene at a time.
Neuroscientists today generally take that single-gene approach rather than looking at multi-gene combinations, he comments, “because we simply don’t know where to start.”
Picture perfect
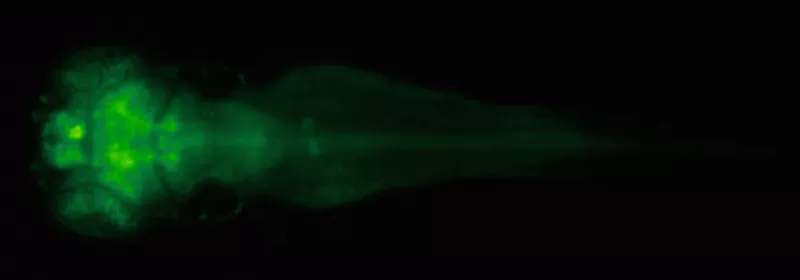
The NIBR team—in collaboration with scientists at the Friedrich Miescher Institute for Biomedical Research, Harvard and MIT—is pursuing two overlapping big-data imaging projects, both in live zebrafish.
One project looks at patterns of activity in the zebrafish brain as the animal is exposed to stimuli. For example, the scientists can display “movies” to fish in a dish and see how their tiny brains react, and go on to see how and where these “neural patterns” may differ between normal fish and fish that have had a suspect gene knocked out. Importantly, the researchers also can search for differences in these neural patterns after the fish is exposed to a drug compound.
This work benefits from a remarkable property of the zebrafish: It is close to transparent when young, which makes it perfect for in vivo imaging. “Basically you can see every organ, every cell in the fish, which is pretty amazing,” Zhu says.
Gerald Sun, a postdoctoral researcher in the Zhu lab, does fish imaging with a powerful microscope in a darkened lab. The “two-photon” microscope picks up signs of calcium flow that indicate neural activity, and produces strikingly detailed and rapid images of the fish’s fraction-of-a-pinhead-size brain.
This setup lets Sun record the activity of just about every individual neuron in the brain, and then examine how the patterns of neural activity reflect given stimuli. He then can compare this neural activity in normal fish brains and brains missing a certain gene or brains treated with a compound.
When behavior breaks bad
The Zhu group’s second major initiative examines the ways in which genetic mutations affect the social behaviors of zebrafish swimming freely around tanks.
This approach works because zebrafish display a variety of quantifiable social behaviors—for example, they tend to interact with each other by shoaling, whereby they swim together, but not necessarily in a synchronized fashion.
To study their social give and take, scientists gather hours of film recording the location of each fish and its body activities with extremely high resolution. Machine-learning algorithms then analyze this onslaught of images.
“We know the orientation of every fish,” Zhu says. “We can basically ask questions such as, ‘When will the two fish be facing each other, head to head? How many times do they do this, where and when, and in what sequence?’ Machine learning can detect the behavioral norm and then flag aberrations in very large data sets across huge populations.”
And once again, the scientists can compare the behavior of normal fish with that of fish lacking certain genes, who may show avoidance behaviors, for instance.
Circuitry in common
But as all these data are gathered, how will their analyses apply to human neurological conditions?
Many human genes associated with autism and schizophrenia cause alterations in nerve synapses, which lead to changes in how sensory information is handled, which then can produce effects such as heightened sensitivity to background noise. Zebrafish with those mutations may also display similar changes in nerve synapses, and the scientists can follow how compounds affect these changes either in fish brain functions or in behaviors, Zhu says.
In addition to testing compounds, zebrafish models may help to clarify the underlying mechanism of approved drugs, including antipsychotic compounds. “We know what these molecules look like and what they bind to, but precisely how they affect brain circuits and brain function, and have their beneficial effects, is a complete mystery,” says Sun. “If we can understand how these drugs work, then we might be able to make better ones.”
Despite all the diversity across vertebrate species, “there’s extraordinary convergence in the types of behaviors and actions we have,” he adds. “They may be handled in different places in the brain but the actual computation may be exactly the same. At the end of the day, the information is all in how the neurons encode information and how they fire together in circuits and networks. We’re confident that the neural circuit principles that we find in zebrafish will generalize to humans.”